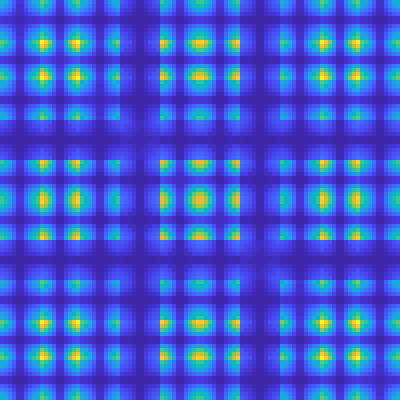
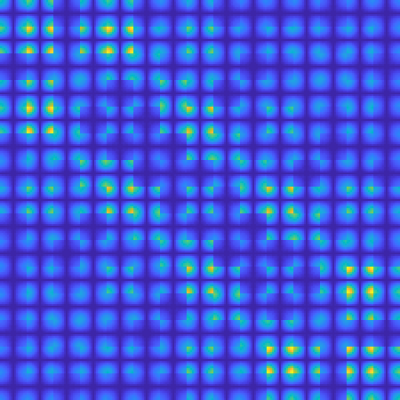
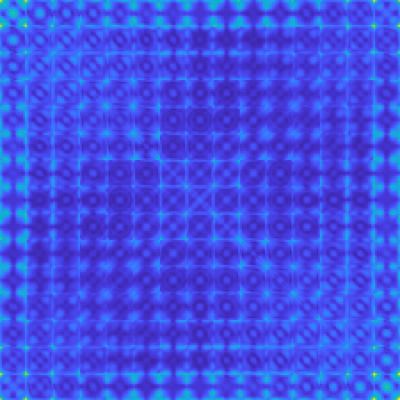
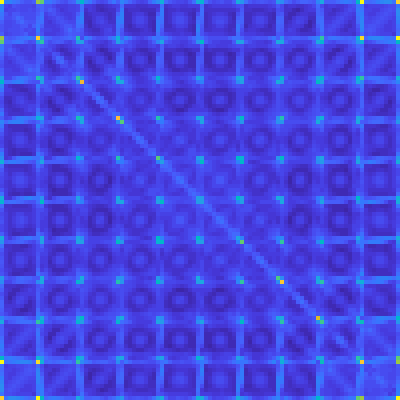
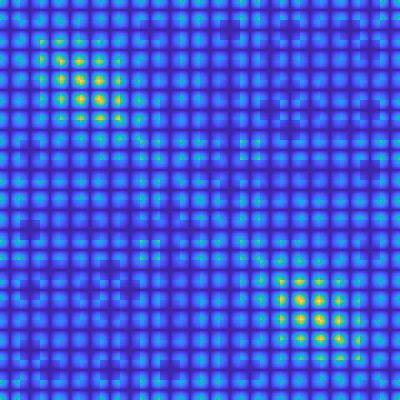
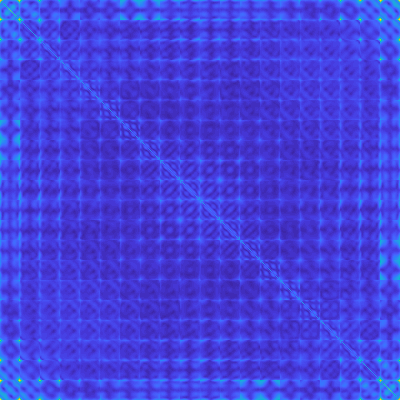
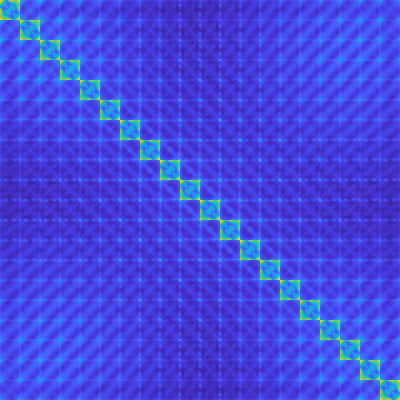
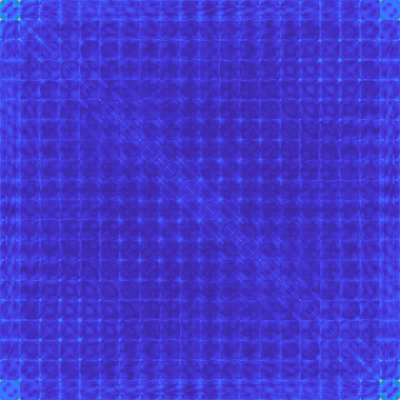
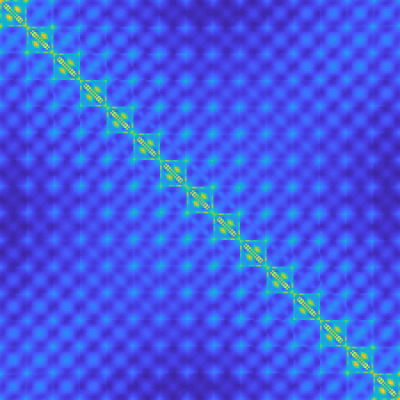
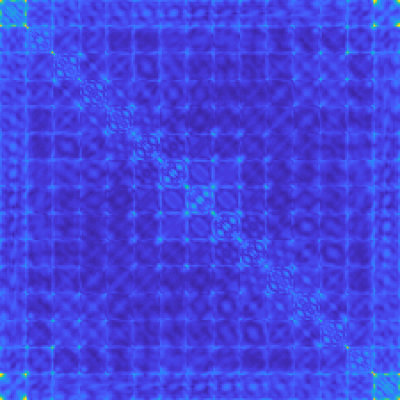
Macromodeling has been and is currently one of the main research activities of our group. The objective is providing the designers of electronic systems with accurate and efficient models of various types of structures, including connectors, packages, transitions, junctions and discontinuities, vias on printed circuit boards, transmission lines, up to complete transmission channels or power distribution networks. All these structures have a significant influence on voltage and current signals, and their parasitic effects must be carefully represented through efficient models. Although several modeling strategies are available even in commercial CAD tools, they result often inadequate for the extended bandwidth required by the ever-increasing clock frequencies, now well into the multi-GHz range. Our research activity is focused on the development of efficient macromodeling tools and algorithms, leading to mathematical/circuit models characterized by a good accuracy and by a reduced complexity.
Modeling of 3D interconnects like connectors, junctions, packages, and vias is one of the most challenging tasks in system-level characterization of high-speed electronic systems. Such structures behave as short circuits at low frequencies, but have a significant influence on the signals with the high data rates that are currently used for digital transmission. A careful Signal Integrity analysis requires accurate macromodels for all these structures. However, due to the 3D nature with complex geometry/material configurations, the modeling procedure must rely on full-wave characterization or on direct measurement. This research activity aims at translating the large amount of data (usually port responses in time or frequency domain) that is obtained by either process into macromodels characterized by low complexity and good accuracy. These macromodels should be available in a form which is directly usable within standard SPICE-like circuit solvers. Several approaches have been followed to reach this goal, with the well-known Vector Fitting algorithm playing a key role.
There are many situations in which a physical structure under modeling is not fully defined. For instance, when a system is still in its design phase, some parameters are unknown and should be determined based on performance optimization. Another situation occurs when some physical parameters cannot be determined a priori, since intrinsically affected by variability. In these situations, it is very desirable to perform optimization or sensitivity analyses through reduced-order compact macromodels which embed in closed form some dependence on these external parameters. These multivariate or parameterized macromodels has been deeply investigated, and a set of robust algorithms based on various formulations of the so-called Parameterized Sanathanan-Koerner iteration have been produced. Recent results show how uniformly stable and passive models can be reliably identified from sampled frequency responses.
Transmission lines structures at chip, multichip, package, and board level constitute one of the most critical parts for the signal integrity of all electronic systems. Nonetheless, an accurate and efficient transient simulation of transmission lines is still a challenging task even in the most advanced circuit solvers. This is due to the intrinsic difficulties in the design of stable algorithms for the time-domain analysis of structures with frequency-dependent parameters. Indeed, it is well known that accurate interconnect models must take into account metal and dielectric losses, which lead to possibly large attenuation at increasing frequency. The underlying physics is best captured using a frequency-domain approach, leading to constitutive parameters with a complex dependence on frequency. A robust approximation is therefore required for the conversion to time domain of the constitutive line equations and the subsequent generation of a line macromodel to be employed in a transient simulation. This research activity is focused on the transmission-line macromodeling based on the Generalized Method of Characteristics (MoC) as well as more general delay-extraction techniques combined with rational approximation in the complex frequency domain. A success story of this research line is the inclusion of a proprietary transmission-line modeling and simulation algorithm denoted TOPLine in the IBM PowerSpice solver.
Standard macromodeling procedures adopt some kind of rational approximation in order to synthesize lumped electrical equivalents for given components or interconnect structures. Depending on the particular approximation process being adopted, the macromodel may result passive or non-passive. Passivity may be defined in a loose sense as the inability of a given structure to generate energy. Non-passive models may lead to instabilities in transient simulations, depending on the termination networks being adopted. This situation can be avoided by checking the models for passivity and by trying to compensate the passivity violations. Our research in this field led to investigate the spectral structure of so-called Hamiltonian matrices associated to the macromodels. The algorithms and tools that we have developed allow to pinpoint all the passivity violations and to eliminate them by iterative spectral perturbations. Hamiltonian passivity characterization is now a widespread technique in several commercial CAD tools.
As opposed to the macromodeling approaches from sampled port responses, other important techniques are available. In particular, if a large-scale circuit or state-space description of the system under investigation is available, classical Model Order Reduction techniques can be applied to compress the model by removing redundant states, hence providing more compact yet accuracy-controlled models. Our research group is active in research and education in this field, by offering a PhD-level course titled “Surrogate and compact modeling: theory for the User”.
The transmission of digital signals always requires at least one driver and one receiver. The characteristics of these devices depend on the application and on the technology. However, they are always made of transistors and built in integrated circuits, and therefore are nonlinear and dynamic elements when looked at their interface ports. Unfortunately, the nonlinear and dynamic effects are important for signal propagation, since any realistic Signal Integrity analysis must take them into account. Accurate transistor-level models in standard circuit simulation environment like SPICE are usually available to designers, but unfortunately these models are so complex that they result practically unusable for systematic analyses. This is the reason why our group has dedicated much efforts in the development of accurate and efficient macromodels for drivers and receivers, including all relevant effects for Signal Integrity issues. A success story of this research line is the development of the tool MpiLog and its deployment within leading semiconductor companies.